Whilst cerebrospinal fluid (CSF) is not as accessible as plasma, it may be invaluable in the search for biomarkers for neurodegenerative diseases and central nervous system (CNS) cancers, and for monitoring CNS-related side effects of certain therapeutics (e.g., for HIV patients). Extracellular vesicles (EVs) are nano-sized, lipid-encapsulated vesicles containing proteins, RNAs and small molecules from their cell of origin. Due to this precious cargo, there is significant interest in investigating EVs in CSF in the search for brain-derived biomarkers in disease. In this article we will discuss the composition of CSF and its implications for EV isolation methods. Plus, we will dive into the research regarding CSF EVs and discuss opportunities for improvement in the field.
The composition of CSF
CSF surrounds the brain and spinal cord, providing mechanical protection whilst also facilitating cell-cell communication and the maintenance of CNS homeostasis. The composition of CSF differs greatly from that of plasma (Table 1), with CSF having very low levels of soluble protein. 1,2 The concentration of EVs is also substantially lower in CSF3, which may be an unexpected advantage. The relative partitioning of CSF from the rest of the body reduces the sources of EVs present in this biofluid, allowing brain-derived EVs to dominate. Whilst knowledge on the proportion of brain-derived EVs in CSF is limited by the lack of good markers, one study found that 16.1% of brain-specific proteins in CSF EVs were neuronal, with 83.9% being glial in origin. 4
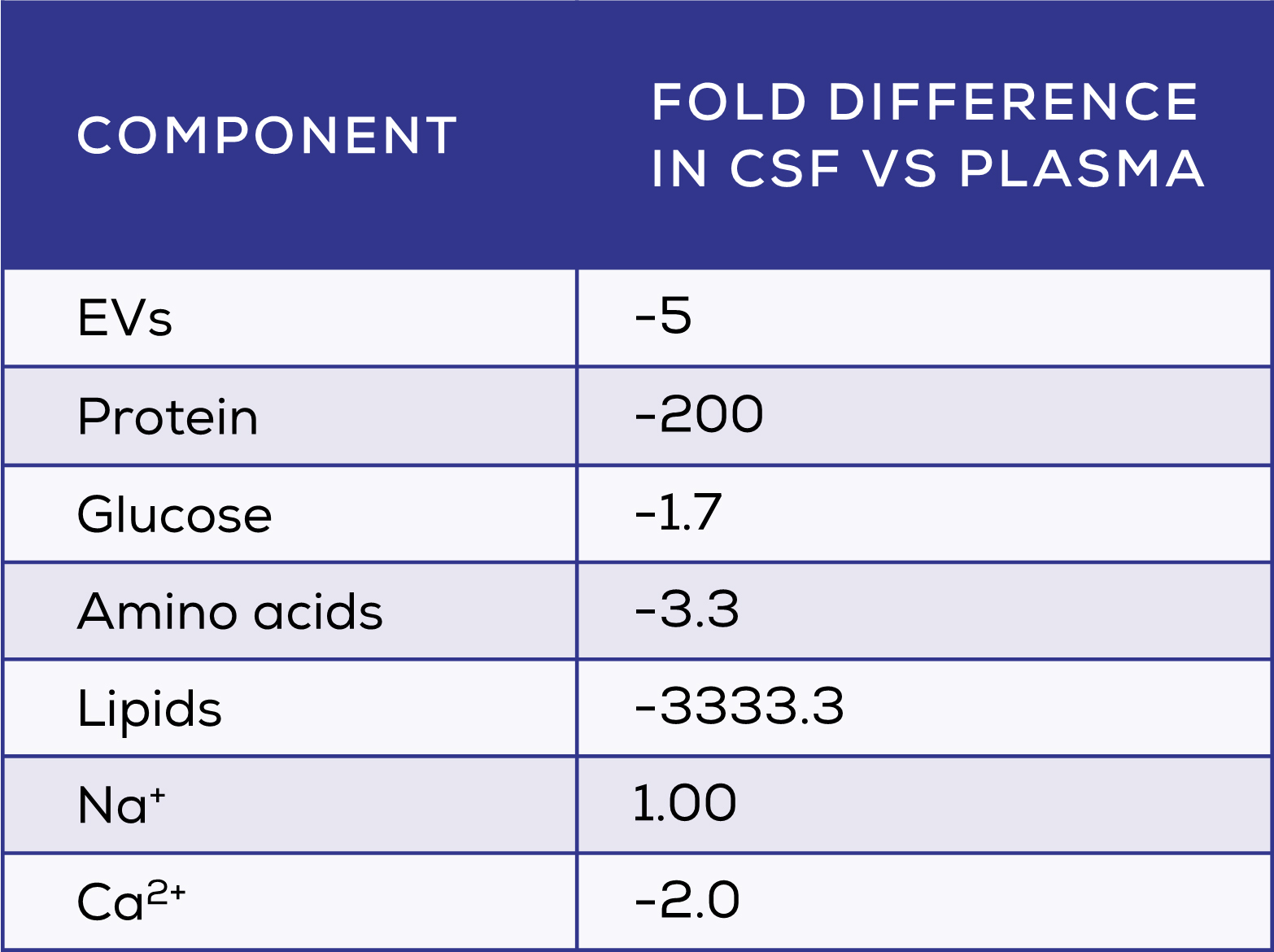
Special considerations for the isolation of EVs from CSF
The challenges associated with isolation of EVs from CSF are different to those for other biofluids. For example, ultracentrifugation (UC) isolates a large number of particles from plasma – though not purely EVs and not without damage to their functionality – but fails to isolate many EVs from CSF. 3,5 In contrast, size exclusion chromatography (SEC)-based qEV columns (qEV 35 nm Series) have been shown to efficiently recover EVs from CSF5.
Another important consideration is sample volume, which is particularly low for CSF samples – yet relatively larger volumes are required by many EV isolation techniques. If a low volume is used in UC, tubes collapse and the sample is lost. This necessitates dilution of small samples, causing a significant decrease in EV number within the diluted sample, even when accounting for dilution factor. 6 This means that some EVs may ‘disappear’ during the dilution process; how this occurs is yet unknown, but may include binding of EVs to the additional plastics (e.g. pipette tips and tubes) used in the dilution process. In CSF where EVs are already at a lower concentration than in other biofluids, minimising EV loss during processing is especially important. For this, techniques which avoid pre-isolation dilution, such as those optimised for small volumes, are ideal. Of note, qEV columns come in a range of sizes to suit all sample volumes from 150 μL with the qEVsingle, to the very largest qEV100 is optimised to isolate EVs from volumes as large as 100 mL (Table 2).

Another method of EV isolation is immunocapture using an antibody directed to an EV surface protein. Purity for immunocapture is usually good, but yield is often very low. 5 However, EVs are heterogeneous by their nature, having different surface proteins and carrying them at different levels. Thus, using immunocapture will only ever isolate a subset of EVs. Even if you were aiming for EVs from a specific cell, there is no guarantee that all EVs from that cell will carry a particular marker, meaning that they may be left behind – and therefore not included in the analysis. The neuronal protein against which many antibodies are directed in the field is L1CAM. Recent evidence has called this method into question, sparking intense debate in the field. The evidence for and against the usefulness of L1CAM as a marker of neuronal EVs for immunocapture has been covered extensively, and the debate is ongoing. Overall, whilst immunocapture can be somewhat specific, it is neither the most efficient nor reliable technique. SEC remains the best all-around choice for isolating a broad range of pure EVs from CSF. 5
The possible medical importance of CSF EVs
Diseases of the CNS are highly complex. They are fundamentally difficult to diagnose and monitor, and further studies are needed to unravel their pathogenesis. This makes research into CSF EVs a potentially fruitful endeavour in these lines of research. However, to date the quality of EV research in CSF lags behind that of other biofluids. Many CSF studies do not isolate their “EVs” but analyse straight from CSF. Others use DIY techniques which are not validated (e.g. progressive filtering down to a 0.1 μm pore size with no soluble protein removal7). There is also very limited to no adherence to MISEV guidelines in many of these studies, which may explain the lack of concordant results in CSF EV research.

Key questions for the future of CSF EV research
The medical importance of good research into CSF EVs is significant due to the severity of the CNS neurodegenerative and cancerous pathologies for which CSF EVs are potential biomarkers. There remain several key questions and areas of concern for the field that must be addressed to improve the quality of research and increase the likelihood of identifying viable biomarkers and pathological mechanisms.
- Can the CSF EV field agree to improve EV isolation and characterisation methodologies? Currently the quality of EV isolation methods in the field is particularly poor compared to those being used in other biofluids. They are also highly heterogenous, meaning that different subpopulations of EVs and contaminants are isolated in different studies, contributing to the lack of concordance between studies. Characterisation of EVs is similarly poor, with many papers – even recently published papers – not including any or very little EV characterisation. Research in the field could benefit from following MISEV criteria for EV isolation and characterisation.
- Are CSF EV biomarkers superior to plasma biomarkers? Given the comparative invasiveness and reduced sample quantity of CSF samples as compared to plasma samples, CSF EV-based biomarkers have unique disadvantages. It is imperative to avoid potentially unwarranted invasive procedures for patients. Comparison with plasma EVs is, therefore, an important step for any potential CSF-derived EV biomarker.
- What is the stability of EVs stored in CSF? CSF for biomarker studies is frequently stored for varying and unreported timescales before EV isolation. Comprehensive studies on the impact of storage on EVs within CSF is required to determine the impact of this practice on the results of studies using these samples.
Addressing these questions will improve the quality of research in the CSF EV research field, which can only be beneficial for both researchers and patients. This also means that there is significant opportunity for high-quality studies in this area to produce novel and important results.
Related article: Chasing L1CAM: A Marker of Brain-Derived Extracellular Vesicles or a Red Herring?