Stem cells, with their innate ability for self-renewal and potency, have long captured the attention of medical science. But recently, with challenges surrounding stem cell survival post-transplantation,1 focus has shifted to their secretome. Enter stem cell extracellular vesicles (EVs): nano-sized vesicles teeming with diverse proteins, nucleic acids, and small molecules. Acting as cellular communicators, they have immense therapeutic potential.
However, the path is not without hurdles. While generating stem cell EVs is feasible, achieving therapeutic-grade purity during isolation requires an effective, reproducible and scalable approach. Our qEV isolation columns range from the qEVsingle for 150 µL loading volumes to customised columns accommodating over 2,000 L as part of qEV PurePath for Therapeutics, meaning that we can support you no matter what stage you are at in bringing your product to market.
Explore the work of fellow stem cell EV researchers who have entrusted their EV isolation to our qEV columns.
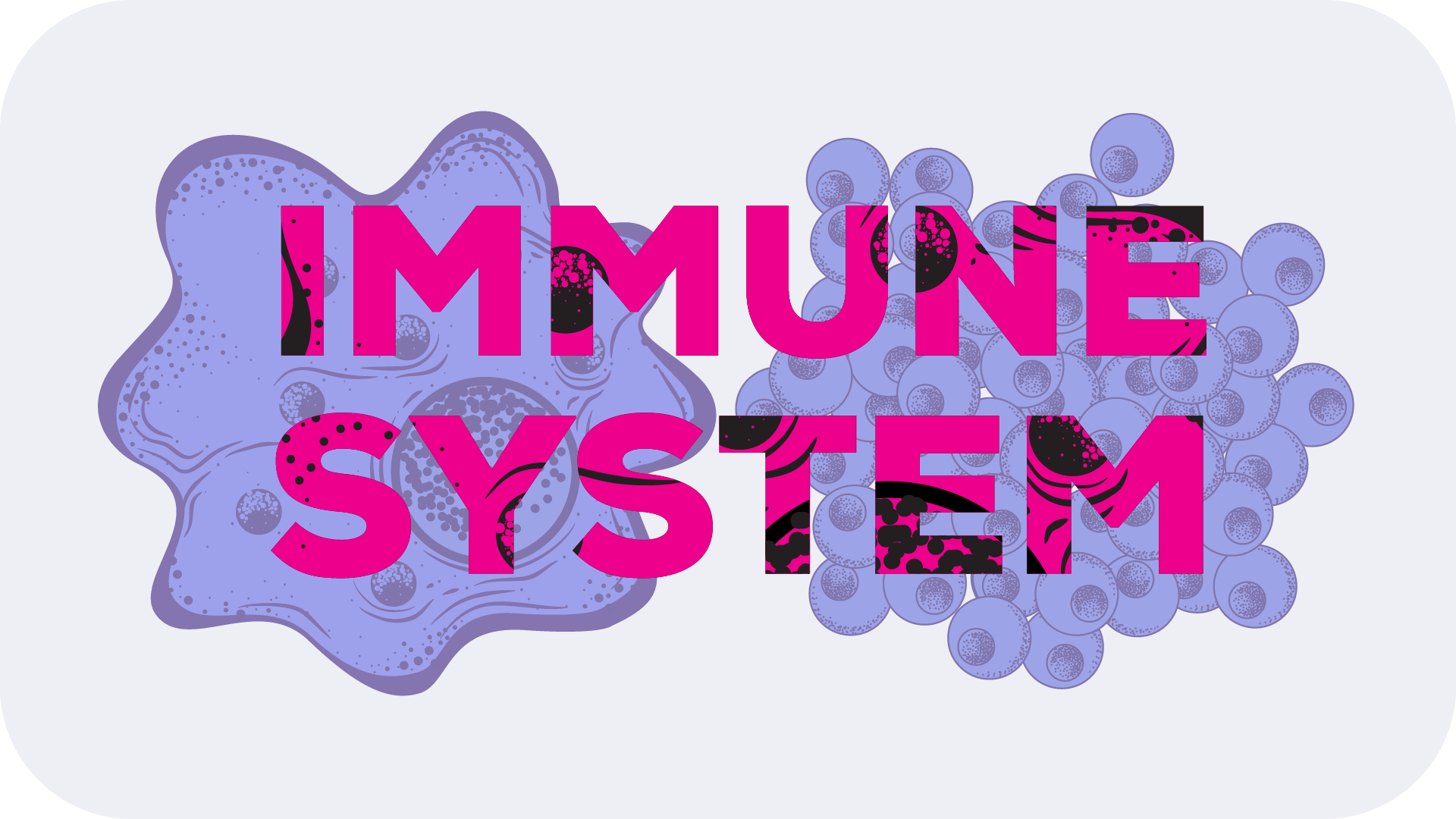
qEV columns, stem cell EVs and the immune system
Symonds et al., (2023). Adipose derived stem cell extracellular vesicles modulate primary human macrophages to an anti-inflammatory phenotype in vitro.
Adipose tissue stem cell EVs, like their counterparts, are known to enhance tissue repair and regeneration.2 Symonds et al., (2023)3 hypothesised that these EVs foster an anti-inflammatory setting, especially crucial for successful autologous fat grafting after mastectomy, a procedure often hindered by inflammation. Utilising the qEV10 35 nm Series for EV isolation, they treated macrophages with these EVs. High-dimensional flow cytometry revealed changes in pro-inflammatory M1 and neutral M0 macrophage subtypes, while anti-inflammatory M2 remained unaffected. This hints at a targeted alteration, potentially benefiting procedures like autologous fat grafting.
Zhang et al., (2020). Mesenchymal stem cell-derived exosome-educated macrophages alleviate systemic lupus erythematosus by promoting efferocytosis and recruitment of IL-17+ regulatory T cell.
Macrophages play a pivotal role in efferocytosis, or the clearance of apoptotic cells.4 In systemic lupus erythematosus (SLE), this process is impaired, leading to apoptotic cell accumulation and adding to SLE's immunopathology.5 Zhang et al., (2022)6 investigated if bone marrow mesenchymal stem cell (MSC) EVs, known to influence macrophage function7, could mitigate SLE inflammation. They treated SLE mouse models with MSC-derived EVs (isolated using qEV columns) and noted a decrease in SLE nephritis. This may be attributed to a shift from pro- to anti-inflammatory renal macrophages, promoting efferocytosis. These findings pave the way for exploring MSC EVs as potential SLE treatments.
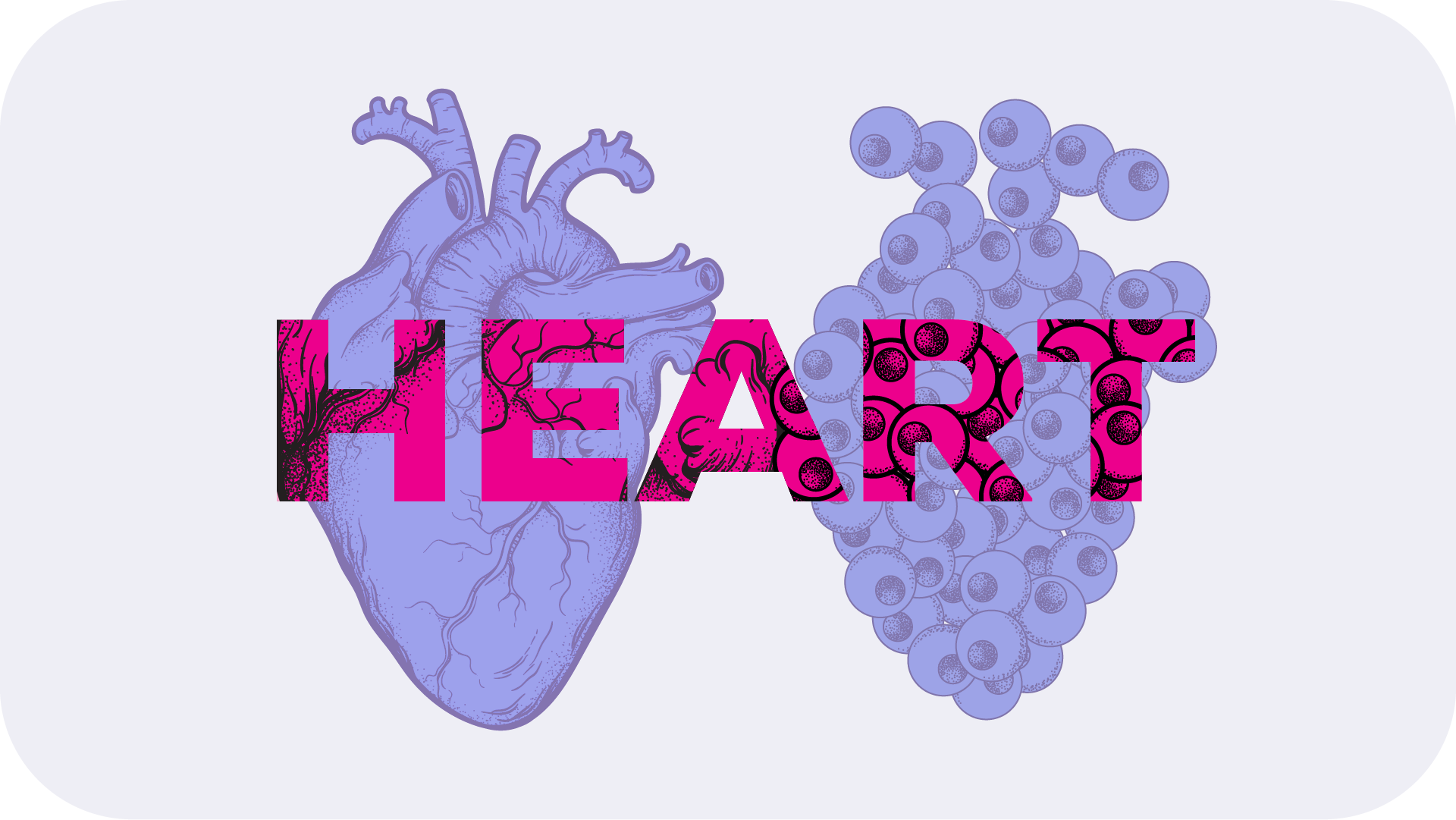
qEV columns, stem cell EVs and the heart
Xuan et al., (2020). Extracellular vesicles from notch activated cardiac mesenchymal stem cells promote myocyte proliferation and neovasculogenesis.
MSCs from the heart may play a role in vascular restructuring post-myocardial infarction.8 Their differentiation, believed to hinge on cell-cell contact and the Notch1 pathway9, was examined by Xuan et al., (2020).10 They manipulated Notch1 expression in cardiac MSCs and studied the therapeutic potential of their EVs (isolated with qEV columns) in a cardiac injury mouse model. While standard cardiac MSC EVs enhanced cardiac function post-injury, this was amplified with Notch1 overexpression. In contrast, Notch1-suppressed EVs had no positive effect. In vitro findings suggested these benefits arose from increased cell migration, tube formation, and viability, mirroring in vivo observations of improved vascular density and diminished fibrosis. Hence, cardiac MSC EVs, particularly from Notch1-overexpressed MSCs, emerge as promising post-infarction treatments.
Jiang et al., (2022). IL-10 partly mediates the ability of MSC-derived extracellular vesicles to attenuate myocardial damage in experimental metabolic renovascular hypertension.
Effective treatments for renovascular hypertension remain elusive. Past studies demonstrated that adipose MSC EVs, possibly influenced by IL-10, ameliorated this condition in pigs.11,12 Jiang et al., (2022) delved into whether adipose MSCs, particularly their IL-10 component, would be effective even when hypertension was accompanied by metabolic syndrome. Utilising qEV columns, they isolated EVs from these MSCs, with some undergoing IL-10 knockdown. Notably, they detected IL-10 mRNA within the MSC EVs. While the standard MSC EVs rectified the M1/M2 macrophage balance and fostered an anti-inflammatory environment, the IL-10 deficient EVs did not. They also noted reduced cardiac fibrosis and enhanced angiogenesis. The connection between immune modulation and these cardiac improvements warrants further exploration.
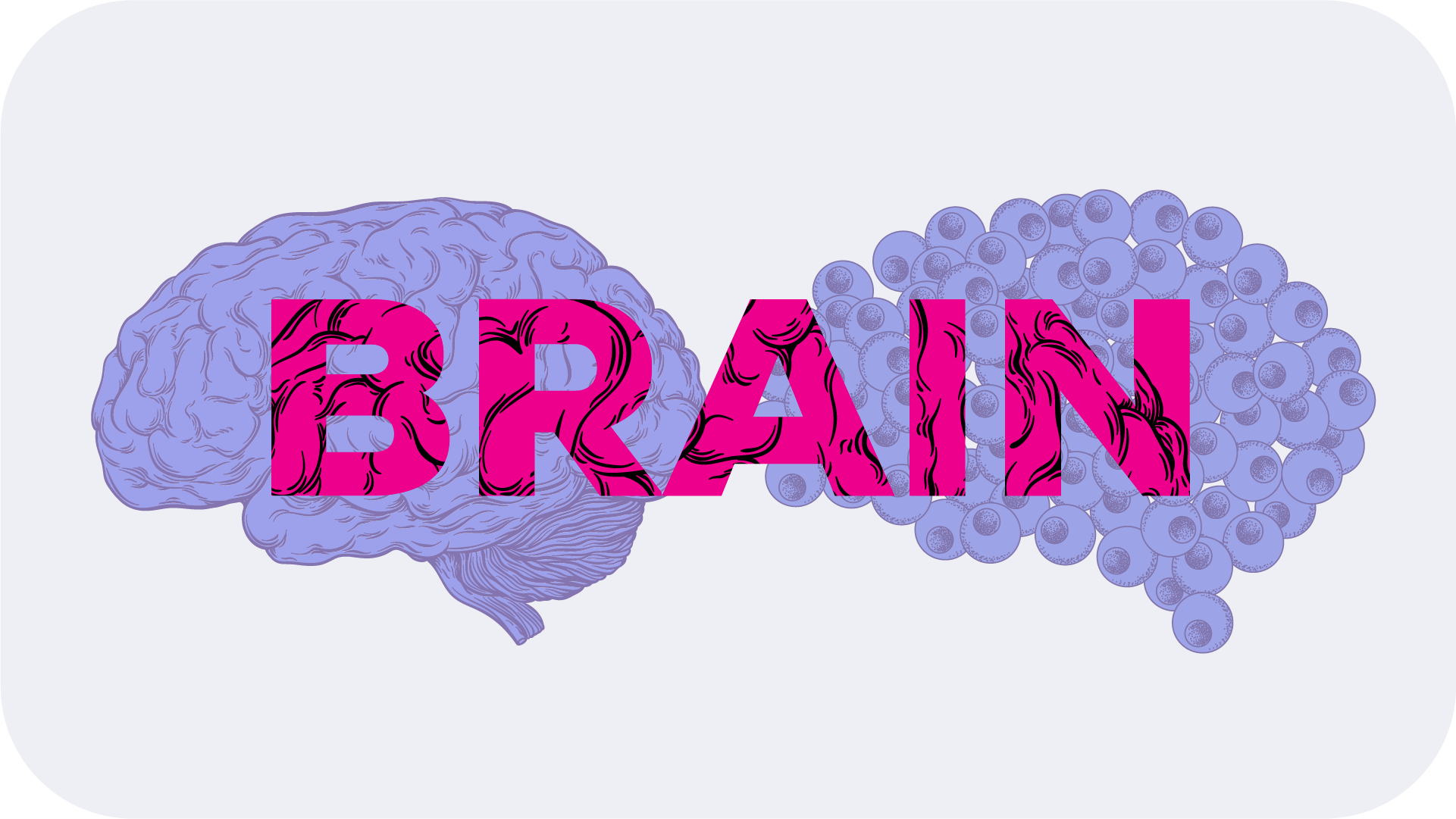
qEV columns, stem cell EVs and the brain
Ocaña et al,. (2023). Neural stem cell-derived extracellular vesicles favour neuronal differentiation and plasticity under stress conditions.
The potential of neural stem cells in treating neurodegenerative diseases and neural injuries has been tempered by reports indicating a mere 1% cell survival 4 weeks post-transplantation.1 Ocaña et al,. (2023)13 aimed to study the influence of neural stem cell EVs — extracted via qEVoriginal columns — on existing neural cells in vitro. Their findings revealed enhanced proliferation and neurite length in standard and oxidative stress settings, but not during inflammation. Across all conditions, neural stem cell EVs bolstered neurite spine numbers and rejuvenated dysfunctional neurons. While these findings hint at the therapeutic promise of neural stem cell EVs in specific scenarios, further studies are needed to validate them as potential treatments for neural diseases.
Bertolini et al., (2020). Interplay between V-ATPase G1 and small EV-miRNAs modulates ERK1/2 activation in GBM stem cells and nonneoplastic milieu.
Local tumour recurrence is common in glioblastoma, with surgery the only current offering for a hope of a cure. In this case, glioblastoma stem cells and their EVs could be the villains, especially those with higher V-ATPase G1 levels14. Bertolini et al., (2020)15 sought to investigate this using patient cultures that had either high (V1G1high) or low (V1G1low) in V-ATPase G1 expression. These EVs, isolated using qEV columns, were added to low grade glioma cells. The V1G1high EVs were internalised to a greater degree than V1G1low and also increased culture survival time, proliferation and migration. These effects were mitigated by a V-ATPase G1 inhibitor, indicating a potential therapeutic direction.
qEV columns: ensuring purity in EV research
In the quest to understand the role of a cell's secretome, the purity of EV samples becomes paramount. A tainted sample can skew results, potentially misattributing effects to EVs when instead contaminants were behind the effect. Prioritising isolation methods that emphasise purity is essential, particularly when exploring the potential of EV-based therapeutics. The qEV isolation platform, with its automation capabilities, has gained traction in the stem cell EV domain precisely for this reason. If you're keen to delve deeper into qEV columns or our bespoke service, qEV PurePath for Therapeutics, we invite you to discuss your research aspirations with us.
Get in touch now to elevate the clarity of your stem cell EV research.